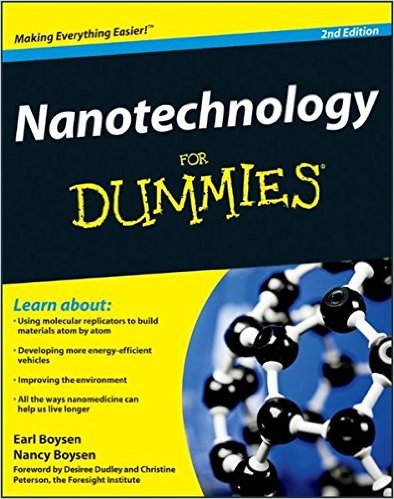
Nanotechnology For Dummies – by Earl Boysen and Richard Booker
ISBN: 0470891912 READ: July 2016
Packed with info about hundreds of topics and subtopics on Nanotechnology. I found it a little hard to get through. The middle especially felt like it dragged and many of the topics were repeated throughout the book. The book was mostly an overview of the topic and did not go deep into how nanotech really works. I would recommend for someone who is looking for a broad understanding about how nano is/will be impacting our lives… Spoiler: Its impacting us in any way you can think of.
PART 1 : NANOTECHNOLOGY BASICS
Nanotechnology is the study and use of structures between 1 nanometer (nm) and 100 nanometers in size.
Imagine you need to build the tiniest computer chip possible. Using the bottom-up approach, you would use nanotechnology to assemble the chip atom by atom, placing each type of atom in a specific location to build the circuit. With the top-down approach, you would instead create the computer chip by carving away at bulk martial – much like a sculptor – to create nanosized features, never dealing with the atomic level of matter.
TEM: Transmission electron microscope. This device has sever hundred times the power of a traditional microscope, which uses light for magnification. A TEM uses a focused beam of electrons, instead of light, to pass through and magnify an object by a factor of up to about 1000000.
Feynman’s speech, in which he asked, “What would happen if we could arrange the atoms one by one the way we want them?, did a good job sating the possibilities of the nanoscale world.
IBM Zurich invented a machine named the scanning tunneling microscope (STM), which can move objects at the atomic scale.
Even though much has been accomplished in developing nanotechnology applications, much more is still deemed possible. Three of the most fascinating examples – all in the speculative phase – are the space elevator, molecular manufacturing, and cellular repair.
Bucky Balls
Also called fullerenes, were one of the first nanoparticles discovered. Buckyballs are composed of carbon atoms linked to three other carbon atoms by covalent bonds. However, the carbon atoms are connected in the same pattern of hexagons and pentagons you fin on a soccer ball, giving a buckyball a spherical structure.
The most common buckyball contains 60 carbon atoms and is sometimes called C60
Carbon Nanotubes
Another significant nanoparticle discovery that came to light in 1991 was carbon nanotubes. They are strong and not brittle. They can be bent, and when released they will spring back to their original shape.
Carbon atoms have a natural capacity to form covalent bonds with many other elements because of a property called electronegativity. Electronegativity is a measure of how strongly an atom hold onto electrons orbiting about it. Because carbon has an electronegativity in the middle of the range, it can form stable covalent bonds with a large number of elements.
Graphene
Although carbon can form 3d lattices by bonding with four other carbon atoms to form diamond, it can also form 2d sheets which it bonds to three other carbon atoms. These sheets are called graphene.
Printing patterns with nano-lithography
The smallest structure (called the minimum feature size) on the microprocessors Intel is currently shipping is 32 nanometers in width (2011).
To create nano sized features for integrated circuits on silicon wafers requires a machine called a stepper, which uses a technique called lithography to print a pattern on the chip.
PART 2 : NANO APPLICATIONS
Seeking Smaller Chips
There are some limitations to how smaller minimum feature size the top-down method can produce. As researchers keep finding innovative ways to make the minimum feature size smaller and smaller, and to increase the number of transistors per chip, they will someday hit a wall. At some point below a minimum feature size of 10nm, instead of using the top-down method of etching away silicon, it’ll probably be necessary to use nanoparticles of the desired size to form these minimum features; or it may even be necessary to use bottom-up methods to build part of all of the integrated circuit from individual atoms or molecules
Here’s how the flash transistor works as a memory device
– When electrons are stored on the floating gate, they stay there, even if the power is turned off, because the floating gate is surrounded by an insulating material that traps electrons.
– Electrons on the floating gate repel electrons from the surface of the channel between the source and the drain, preventing current from flowing through the transistor.
– When the computer reads data from the flash memory, it reads ones or zeroes.
Making Displays Flexible
The display in your cell phone and computer includes layers or transparent, conductive material called indium in oxide. These layers of indium tin oxide have two problems: This material is expensive, and it cracks if you flex it.
Taking Advantage of Quantum Dots
To excite the fluorescent dots used in many displays, you need to shoot electrons at pixels. Quantum dot displays, on the other hand, use an electric field to excite electrons and generate light. You control the light generated in each pixel by using electrode to apply a voltage.
Detecting All Kinds of Things with Nanosensors
Nanotechnology can enable sensors to detect very small amounts of chemical vapors.
Because of the small size of nanotubes, nanowires, and nanoparticles, a few gas molecules are sufficient to change the electrical properties of the sensing elements.
Potential of Nanosensors:
– Air quality monitoring
– Cell phones: a food science worker out in the field could wave a cell phone over a head of lettuce and have the device sense the presence of salmonella or dangerous levels of pesticides.
– Contagious airborne diseases: You could use nanosensors to scan people boarding -lanes to stop disease carriers from travelling and reduce the spread of pandemic contagions.
Making Tennis Balls That Bounce Longer
The hiss when you open a new can of tennis balls is because the can is full of pressurized air. After the can is open the pressurized air inside the tennis balls will slowly leak out until the pressure inside the ball equals the air pressure and the balls lose their bounce. If you’ve ever hit the tennis courts, you know that a sluggish ball is a real downer. Wilson has introduced a Double Core technology in their tennis balls that uses a nanocomposite coating that makes the balls bounce twice as long as other balls. The coating used the these balls is a mix of rubber and nanoclay particles that provides a gas barrier that slows the the loss of pressurized air from the call. This nanocomposite material allow you to use the ball longer at full performance.
Changing Food Characteristics with Nanomaterials
Nanoparticles are being used to deliver vitamins or other nutrients in food and beverages without affecting their taste or appearance. These nanoparticles encapsulate the nutrients and carry them through the stomach into the bloodstream.
Of all the things that nanotechnology makes possible, the promise of making us live longer and healthier lives is probably the most intriguing to many of us. Nanotechnology may be able to extend our live in two ways. one is by helping to eradicate life threatening diseases such as cancer, and the other is by repairing damage to our bodies at the cellular level.
Magnetic Resonance Imaging (MRI)
Most of the molecules in your body contain hydrogen. Water molecules have two hydrogen atoms, and the organic molecules that make up the rest of our bodies are called hydrocarbons because they contain hydrogen and carbon. The magnetic fields generated by the MRI machine interact with hydrogen throughout the body, producing an image of all the organs.
Protons have spin. The direction of that spin determines the direction of a magnet, which is composed of the spins of all the charged particles (protons and electrons) together.
To take an MRI image, the MRI machine generates a radio frequency pulse that has just the right amount of energy to flip the spin direction of the protons. When the protons flip back to the spin direction aligned with the magnetic fields, they send out another radio frequency pulse. This pulse is detected by the machine, which then uses it to generate an image. The time it takes for the protons to flip back and generate the return radio frequency pulse depends on the protons location in the body and the density of the tissue at that location. This so called relaxation time is different for protons in an organ, such as a kidney, than for protons in the bloodstreams and is different for healthy tissues than it is for cancer tumors. These differences in the relaxation time are used to generate the MRI images.
Delivering Drugs More Efficiently
Cell membranes in our bodies are composed of molecules called phospholipids. One end of these phospholipids, called the head, is hydrophillic, meaning that it mixes well with water. On the other end of the cell are two tails that are hydrophobic, meaning that they don’t mix well with water.
The result of this structure is that the cell membrane blocks the entry of many therapeutic drugs into the interior of the cell, motivating researches to develops methods to deliver these drug molecules through the cell membrane.
Proteins are designed to deliver materials through cell membranes. For example, hemoglobin is a protein that picks up oxygen in your lungs and distributes it to cell throughout your body Hemoglobin is actually capable of burrowing through cell membranes to deliver oxygen. In a different approach to drug delivery, People are enclosing therapeutic drugs in an albumin protein. Nanoparticles of albumin can burrow through cancer call membranes and deliver to the interior.
Another method is a little more aggressive. This method, developed at GIT blasts temporary holes in the cell membranes, allowing drugs to enter the cell. Researchers inject carbon nanoparoticles into the fluid floating around caner cells, and then a laser heats the fluid. This head creates gas bubbles; when the bubbles burst they blow a hole in the membrane.
Sequencing DNA
Determining a person’s DNA involves finding the sequence of the molecules, called bases, which connected single strands of DNA into a double helix. They are: Adenine, thymine, guanine, and cytosine. The order of these molecules determines the genetic structure of an individual. The process of figuring out the order of these molecules is called DNA sequencing. Researchers are developing caster and less expensive methods to do this.
Eric Drexler’s Engines of Creation:
“Aging is fundamentally no different from any other physical disorder; it is no magical effect of calendar dates on a mysterious life-force. Brittle bones, wrinkled skin, low enzyme activities, slow wound healing, poor memory, and the rest all result from damaged molecular machinery, chemical imbalances, and mis-arranged structures. By restoring all the cells and tissues of the body to a youthful structure, repair machines will restore youthful health.”
Big Three of Energy: Generation, Distribution, and Storage
The Inner Workings of a Solar Cell
Light is produced in a bulb by running an electric current through it. A solar cell is just the opposite: light shining on a solar cell produces an electric current.
Understanding How Batteries Work:
Your garden-variety battery has multiple cells, with each cell containing an electolyte, an anode, and a cathode. When you turn on a battery-powered gadget, the voltage between the anode and cathode in the battery causes electrons to flow through the wires to provide power. At the same time, positive ions (atoms that have lost one or more electrons) flow through the electrolyte ( a liquid, paste or film that allows the ions to pass through_ to reach the cathode. The positive ions then combine with electrons and atoms on the cathode to form molecules.
Storing Electrons with Ultracapacitors
Capacitors are like batteries in that they store electrical energy. They simply store electrons on one electrode. Capacitors are ultracapacitors do not have as high a power density as batteries.
Coal burning plants are still the main type of electricity generator in the US, producing as much as 36 percent of our power.
– Use of nanoporous membranes to remove carbon dioxide from power plant smokestacks
– Development of nanomaterials to trap carbon dioxide.
Converting CO2 Into Something Useful
An alternative to sequestration (moving the CO2 underground) is to convert CO2 into a combustible fuel such as methane. All you need to do to make this conversion is to replace the two oxygen atoms with four hydrogen atoms, resulting in CH4 methane.
Cleaning Oil Spills
Researchers at MIT report that they have developed a nanomaterial that can be used to absorb as much as 20 times its eight in oil. “What we found is that we can make paper from an interwoven mesh of nanowires that is able to selectively absorb hydrophobic liquids such as oil from water.
Making Saltwater Drinkable
The idea of making saltwater drinkable is appealing. However the cost of doing so is currently very high.
The revere osmosis process is used in many desalination plants. In this system, you place saltwater under pressure, which forces it through a membrane. The membrane is generally a polymer with many nanosize pores, and is a material that allows water through the pores but stops salts and bacteria.
Researchers are investigating the use of nanomaterials to reduce the pressure needed to force water through the membrane and to reduce the capability of bacteria to grow on the membrane.
Taking Off With The Space Elevator
Another challenge we have to solve before we can roam around space is how to get supplies and people into space affordably.
The space elevator is a device that could provide an alternative way to put things into orbit. Just like the elevator in an office building, the space elevator could be used to transport both materials and people. As with regular elevators, the space elevator will be fitted with a cable. However that cable will have to be strong that any cable you’ve ever seen. Roughly 90000 kilometers long, the space elevator cable will be anchored at the top to an asteroid (called counterweight) in orbit around the earth, and at the bottom by an anchor station, perhaps floating in the ocean. Such a cable will provably be made from carbon nanotubes.
Drafting Nano For Defense
Soldiers clothing doesn’t protect soldiers from bullets to a great enough degree. A nanobattlesuit is being developed that could be as thin as spandex and contain health monitors and comm equipment.
Helping Aircraft Flap Their Wings
Researchers have worked on aircraft that swing their wings in close for high speed flight and extend their wings to provide more list for takeoff and landing.
PART 3 – NANOTECHNOLOGY AND PEOPLE
Living Forever
Perhaps the most exiting possibility for prolonging our lives exists in the potential for repairing our bodies at the cellular level. Nanotechnologists are developing techniques for building nanorobots that would be able to repair damaged DNA, allowing our cells to function optimally.
Cellular repair brings p several ethical questions. If nanotechnolgoy help is to live decades longer, what is the moral imperative for making such benefits available to all? If everybody could live hundreds of years what would happen to our economy and society Would only an elite few get such treatments, and what consequences would that have? If nobody ever died, would people have to stop having children to avoid overpopulation?
University of Waterloo has nano programs, as do many others.
www.nano.uwaterloo.ca
GLOSSARY (Terms I like from the first edition of the book)
adenosine triphosphate (ATP): Organic molecule that stores energy in a biological
cell.
atom: Smallest particle of an element, composed of three types of charged
particles: protons (positive), neutrons (neutral), and electrons (negative).
atomic force microscope (AFM): A scanning probe instrument that measures
the atomic force acting on its tip as it moves along the surface of a sample.
biosensor: A sensor that detects biological molecules such as proteins.
bottom-up fabrication: A construction process that works with the smallest
units of a material first (in this context, atoms) and builds them up into the
form of the final product. Compare top-down fabrication.
buckyball: Short for buckminsterfullerene; a molecule containing 60 carbon
atoms in a soccer-ball orientation. Also known as fullerene or C60.
buckypaper: A randomly oriented network of carbon nanotubes formed into
a flat sheet.
carbon nanotube: A graphite sheet rolled up into a tube.
catalyst: A substance that reduces the amount of energy required during a
chemical reaction. Its presence increases the rate of reaction without the
consuming the catalyst.
covalent bond: Atoms that bond sharing two electrons.
deoxyribonucleic acid (DNA): The nucleic acid that carries the genetic blueprint
for all forms of cellular life.
diffraction: The spreading or bending of light as it passes by an object. An
example of diffraction is using a prism to spread sunlight into a spectrum of
color.
electron-beam lithography (EBL): Fabrication method that uses a tight beam
of electrons to form nano-scale features on a substrate.
entanglement: Relationship in which the quantum states of two or more
objects are always described with reference to each other, even if they’re
physically separate.
fluorescence: A property of some molecules to absorb one wavelength of
light and then emit light at a higher wavelength.
fullerene: A molecule containing 60 carbon atoms in a soccer-ball orientation.
Also known as buckminsterfullerene, buckyball, or C60.
graphite: A flat sheet of benzene rings attached together.
gray goo: Nanotech-disaster scenario in which myriads of self-replicating
nano-assemblers make uncountable copies of themselves and consume the
earth — “gray” because they’re machines; “goo” because their small size
makes them look like a thick liquid when taken together.
hemoglobin: Oxygen-carrying protein in blood cells.
hydrophilic: “Water-loving” materials that are soluble in water. In a molecule,
the part of the molecule that is attracted to water molecules.
hydrophobic: “Water-fearing” materials that do not dissolve in water. In a
molecule, the part of the molecule that is repulsed by water molecules.
impedance: The degree to which a wire resists the flow of electricity.
laser: Acronym for “light amplification through stimulated emission of radiation.”
An intense, powerful beam of light produced by this process is made
up of nearly parallel waves.
microelectromechanical system (MEMS): A mechanical system or machine
that exists at the micro-level.
molecule: Two or more atoms chemically bonded together.
nano: Greek for “dwarf,” meaning one billionth.
nanometer (nm): One billionth of a meter.
nanotechnology: Technology development at the atomic and molecular
range (1 nm to 100 nm) to create and use structures, devices, and systems
that have novel properties because of their small size.
nanowire: Very small wires composed of either metals or semiconductors.
organic molecules: Carbon-based molecules that make up the solid portions
of living things, as well as certain materials such as plastics and oil.
parallel processing: Simultaneous execution of the same task on multiple
processors. Fast, nano-scale processors could make this technique possible
on an unprecedented scale, as in the quantum computer.
phospholipids: Naturally occurring amphiphiles that make up human cell
walls.
photolithography: A computer-processor fabrication technique that uses
light to expose a photosensitive film, resulting in the needed pattern of circuits
at a much smaller scale.
photon: A particle that is a packet of light.
photonics: The science of manipulating photons.
plasma: A gas made of charged particles. An example of naturally occurring
plasma is lightning. You may have seen plasma lamps, glass globes with
sparks shooting around inside.
polymers: Plastic — large molecules made from many smaller molecules usually
composed of carbon atoms bonded in long chains.
quantum computer: A computer that exploits the quantum mechanical
nature of particles, such as electrons or atomic nuclei, to manipulate information
as quantum sized bits (qubit). This quantum computer will be able to
perform quick operations in parallel solving problems that can’t be solved
with today’s computer (for example, factoring large numbers).
quantum cryptography: Cryptography scheme that relies on quantum
mechanics to ensure accurate key exchange and prevent eavesdropping.
quantum dot: A semiconductor nanocrystal that exhibits quantum behavior
in optical or electrical processes.
quantum mechanics: In physics, a theory that describes physical interactions
between atoms more accurately than classical physics, often with
results that seem strange from an everyday frame of reference.
quantum tunneling: A quantum-mechanical effect of transitioning through a
state that classical physics would forbid. An analogy is throwing a ball at a
wall and having it appear on the other side.
quantum: In atomic physics, a discrete and basic unit, similar to the way an
individual electron is the basic unit of electricity. Plural form is quanta.
qubit: Quantum bit — smallest unit in quantum computing.
scanning electron microscope (SEM): Electron microscope that creates
images of nanoscale features by bombarding the surface of a sample with a
stream of electrons, scanning back and forth, and reading the reflected electrons
as they bounce off the surface.
scanning probe microscope: An instrument that studies the properties of
surfaces at the atomic level by scanning an atomically sharp probe over the
sample. This produces an image of the sample’s topography with atomic
resolution.
scanning tunneling microscope (STM): The first scanning probe instrument —
measures electrons tunneling between a scanning tip and a conducting surface.
Schottky barrier: Area of resistance to electrical conduction, occurring at the
junction between the metal wires and the semiconductor in a computer
processor.
semiconductor: Material that has more electrical conductivity than an insulator
(which has no conductivity) but less than a conductor; it can be made to
insulate or conduct electricity in patterns, as in a computer processor.
superconductor: A material through which electricity flows with zero
resistance.
superposition: When an object simultaneously possesses two or more values
of a specified quantity. Useful in the development of quantum computers.
surface tension: The pull of a liquid into its most compact form to minimize
the amount of energy used, keeping the surface area to a minimum.
transistor: A switch that determines whether a bit is a 1 or a 0.
uncertainty principle: In quantum mechanics, a principle made famous by
Werner Heisenberg: Measuring one property in a quantum state will perturb
another property. You can, for example, measure the position or momentum
of an electron — but not both at once.
valence electrons: The electrons in the outermost shell of an atom. These
electrons largely dictate the chemical reactions of the atom.
van der Waals: Weak electrostatic forces between atoms.
viscosity: The measure of resistance of a fluid — its “thickness.”
wavelength: In physics, the distance between one wave peak and the next in
a transmitted wave of radiant energy. Typically measured in nanometers.